48uep6bbphidcol4|ID
48uep6bbphidvals|304
48uep6bbph|2000F98CTab_Articles|Fulltext
Somatostatin was first isolated in the early 1970’s from sheep hypothalamus as a factor inhibiting the secretion of pituitary growth hormone and thyrotropin.[1,2] As the inhibitory effect on the secretion of growth hormone was first described, the peptide was named somatostatin (SS) or somatotropin release-inhibiting factor (SRIF).[1,2,3,4,5,6] Somatostatin receptors (SSTRs) are present in several organ systems and have an important function in the regulation of both endocrine and exocrine secretion.[4,5,6] SSTRs are integral membrane glycoproteins that are described in a variety of tissues throughout the body.[7] SSTR are members of a distinct subfamily of GTP-binding protein-coupled-seven-helix transmembrane spanning receptors.
Somatostatin is a 14-amino acid polypeptide hormone with one di-sulfide bridge between 2 cysteine residues. SSTRs are expressed in the majority of tumors of neuroendocrine origin and in some medullary thyroid cancers. SSTR receptors are transiently expressed during wound healing. So far five SSTR subtypes have been identified and cloned[8]. The very short biologic half-life in blood of somatostatin (2 to 4 minutes) allows little tumor uptake for imaging and is therefore non favorable for imaging. Octreotide, a more stable analogue consisting of 8-amino acids has been developed that is relatively resistant to enzymatic degradation. The modified analog with its longer half-life of 1.5 to 2 hours, has permitted introducing, a therapeutic agent for neuroendocrine tumors (NET) with proved expression of SSRT. Octreotide binds with high affinity to SSTR2and SSTR5 and, to lesser extent, to SSTR3. It does not bind very strongly to SSTR1 or to SSTR4 [9]. The expression of somatostatin receptors in NETs of the intestine, pancreas and lung has led to development of the field of somatostatin receptors targeting in oncology, for both the diagnostic and the therapeutic level.[10]
GEP- NETs represent about 2% of all the GI tumors,[11] but their prevalence has increased substantially over the past three decades, only in part as a consequence of increased awareness and improved diagnostic techniques.[12] The most recent estimates suggest a global clinical incidence of 2.5-5 cases/100,000 per year,[12,13] with an imaging incidence 2-5 times higher than the clinical one, and a slight predominance in females.[14],
The term carcinoid (from the German Karzinoide) was introduced in 1907 by Oberndorfer to identify some ileal tumors, originating from the enterochromaffin cells (EC) that produce serotonin, characterized by a better prognosis in comparison with adenocarcinomas. The term carcinoid should be used to indicate the serotonin-secreting tumors.[16]
GEP NETs arise within the GI tract, but NETs can also occur elsewhere such as in the bronchus and lung (bronchial epithelium), hypophysis, thyroid, parathyroids, thymus, adrenal cortex and medulla, and paraganglia. GEP-NETs can preserve and amplify the activity of the origin cells characterized by secretion of a number of peptides and neurotransmitters, which can lead to the development of typical clinical syndromes by the so called “functioning” tumors, or they can be biologically inactive (“nonfunctioning” tumors).[17,18] GEP NETs are usually sporadic, but they may also be multiple and may occur in some genetic syndromes such as multiple endocrine neoplasia (MEN) type 1, von Hippel Lindau syndrome(VHL), neurofibromatosis type 1 and tuberous sclerosis.[19,20]. Their frequency in these syndromes varies from very low (< 1%) for carcinoid to high (80%-100%) for pancreatic endocrine tumors (insulinomas 5%-20%, gastrinomas 25%-30%, non-functioning > 50%).[21]
Clinical manifestations of GEP NETs are very heterogeneous: indeed, they can either remain asymptomatic for years, or can occur with obstructive symptoms, such as abdominal pain, nausea, vomiting, cholestasis, or can present with metastases, found accidentally, or can occur with typical syndromes due to hormonal hyper secretion. In most cases, because of vagueness of symptoms, the diagnosis is delayed (3-10 years on average), with an increased risk of developing metastases.[22]
The prospect of using radiolabeled receptor-binding somatostatin analogs to target receptor-expressing tissues in vivo offers diagnostic and therapeutic potential in nuclear medicine. Binding to the receptor on the target cell surface generally triggers the signal transduction mechanism of the target cell, and the biologic effect of the ligand is transmitted to the target tissue. The high affinity of the ligand for the receptor promotes retention of the radiolabeled ligand in receptor-expressing tissues, whereas it’s relatively small size facilitates its rapid clearance from the blood stream and nontarget tissues. Tumors may over express various receptortypes depending on their origin.[23]
We will review the most common radionuclide procedures for imaging and therapy for GEP-NETs using nuclear medicine techniques.
Radiolabeled somatostatin analogs
Several somatostatin analogs have been successfully radiolabeled for diagnostic and therapeutic use, and others are under development. Table 1 shows the most common radiolabeld somatostatin analogs used for GEP-NETs. The relative usefulness of these radiolabeld somatostatin analogs is a function of their sensitivities and specificities of receptor binding and the characteristics of the associated radioisotope.
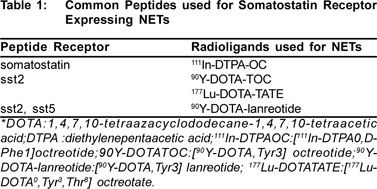
Somatostatin receptor scintigraphy (SRS)
Since there is a very high expression of somatostatin receptors on NET cells, somatostatin receptor scintigraphy (SRS) has improved the imaging of NETs, first with an 123I label and subsequently with an 111In label [24].
Octreotide scintigraphy
Realizing the high diagnostic sensitivity and specificity of octreotide scintigraphy, it was logical to bring this technique to the surgical theater in order to localize neuroendocrine tumors intra-operatively.
Octreotide, substituted N-terminally with DTPA, can be efficiently labeled with 111In. The preferred dose of 111In Octreotide (10 microgram) is about 5 to 6 mci, and planar and SPECT images are obtained with a large-field-of-view gamma camera equipped with a medium-energy parallelhole collimator. The pulse height analyzer windows are centered over the 111In photon peaks (172 and 245 keV) with a window width of 20%.
Imaging is preferably performed 24 hr after injection of radiopharmaceutical. Images at 4 to 6 hr are also recommended because bowel activity is limited, although lesions may be obscured by the relatively high background activity at this time.
Uptake of radioactivity is also observed in the pituitary, salivary gland, thyroid, liver, spleen, kidney, and bladder. Activity is mainly cleared from the circulation by way of the kidney, which is a critical organ from a dosimetry point of view. The overall dosimetry is comparable to that of other 111In labeled radiopharmaceuticals (8-16 mSv).[25]
Most peptide hormone–producing tumors in the pancreas originate from islets cells, but they may also occur in the stomach, duodenum, and the bowel. These include gastrinomas, insulinomas. They can be visualized and localized using 111In DTPA Octreotide.[26] The diagnostic sensitivity of 111 In DTPA-Octreotide scans in patients who have GEP-NETs has been reported to be in the range 80% to 90% , with the highest (100%) in glucagonomas [27,28], 88% in VIPomas, 87% in carcinoids, and 73% in gastrinomas. Sensitivities can be affected by the peptide dose administered, the duration of image acquisition, and the use of SPECT. Failure to visualize some insulinomas may be related to higher-affinity binding for SSTR3 than SSTR2,5.
Adenocarcinomas of the (exocrine) pancreas are typically not detected on octreotide scans.
PET-CT
Because of the enhanced expression of somatostatin receptors, most NETs are visualized by functional imaging with the 111In-labeled somatostatin receptor analog. By identifying previously undetected metastases SRS may instigate a change of management in up to a third of patients.[29] However, the spatial resolution of SRS is markedly poorer than that of CT or MRI; thus, an exact topographic description of pathologic findings is rendered difficult. Positron Emission Tomography (PET) with integrated CT, a modern functional imaging technology, which was shown to be very useful in a variety of malignancies, is able to partly overcome this difficulty. The widely used 18F-FDG is of limited value in NETs because these tumors usually do not show enhanced FDG uptake.[30] PET, however, offers the opportunity to design tracers chemically close or identical to pathophysiologic substrates, which is of great interest in NETs. Table 2 shows the list of PET radiopharmaceuticals used for diagnosis of NETS (available in department of Nuclear Medicine, AIIMS)
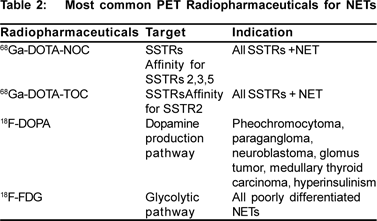
Somatostatin receptor PET-CT with 68Ga-labeled peptides
Positron emission tomography (PET) for imaging somatostatin receptor positive tumours is desirable because of its improved spatial resolution. 68Gallium a generatorproduced positron-emitting isotope is reported to be a promising PET tracer for somatostatin receptor imaging. Labeling of the ligand with 68Ga is easy to perform and generator production of the tracer may ensure its continuous availability.[31]
68Gallium-labeled receptor ligands, such as 68Gallium- DOTATOC, 68Gallium-DOTA-NOC, and 68Gallium-DOTA-TATE which are used in PET and are becoming gold standard of 68Ga-labeled peptides.[32] DOTA-TOC or DOTA-NOC can be easily and quickly labeled with 68Ga, and synthesis result in a product with high radiochemical purity.[33,34] The 68Ga-labeled peptides show a rapid renal clearance and are rapidly accumulated in the tumors (80% within 30 minutes); concentration in tissues without expression of SS receptors is low, providing higher contrast imaging.[35,36] The activity administered in adults is 100-150 MBq (2.7-4 mCi) and imaging is acquired 60 to 90 minutes after injection.68 Gallium-labeled peptides, virtually all well differentiated NETs (WHO 1-2) and other neuroendocrine tumors with high somatostatin receptor density up to a size of a few millimeters can be imaged and precisely localized. The poorly differentiated NETs (WHO 3), which distinguish themselves through a high rate of proliferation and an increased glucose metabolism, can be better detected using 18F-2-deoxy-2-Fluor- D-glucose (FDG) PET/CT. The sensitivity 68Ga-labeled somatostatin analogs PET was approximately 95%, whereas that of SRS was markedly lower—that is, 58%.[37]
PET/CT with 18F-FDOPA
For the PET examination, the patients fasted for a minimum of 2 hours but were well hydrated orally. 18F-FDOPA (300 MBq) was administered intravenously. After an accumulation time of approximately 40 min, PET scan from the brain to the thigh was performed, followed by a CT scan. Visualization of basal ganglia in brain as an in vivo quality control for the 18F-FDOPA. With respect of overall diagnostic performance, 18F-FDOPA PET and SRS had a high specificity of nearly 100%. The sensitivity 18F-FDOPA PET was approximately 85%, whereas that of SRS was markedly lower—that is, 58%.[37,38]
Figure 1,2,3,4 shows the images of NETs from Siemens Biograph-II PET/CT available in department of Nuclear Medicine, All India Institute of Medical Sciences, New Delhi,India.
Prerequisites for somatostatin receptor mediated radiation therapy
A prerequisite for therapy is that the absorbed dose to tumor tissue is considerably higher than the absorbed dose to normal tissue, either due to high uptake or long biological half-life in the tumor tissue. During decay, a radionuclide emits different types of radiation, i.e. electromagnetic radiation (photons) and particles (e.g. electrons). Photons with appropriate energy (70-400 KeV) can be used for scintigraphy, as photons can pass through the body and be detected by a gamma camera. Electrons can be used for therapy and are completely stopped in tissue within their ranges (about 5nm- 1cm) and during retardation the electron transfers its energy to the tissue. Absorbed dose is energy deposited per unit mass. The risk, or in case of therapy, the possibility for tissue damage is proportional to absorbed dose. During certain types of radionuclide decay, auger and conversion electronare emitted. These electrons may have very low energy, but since the range is short, their energy is absorbed within a small volume. The absorbed dose can, therefore, be locally very high. After uptake into the tumor cells (internalization), decay at a position close to the DNA may have potent cytotoxic effects.[39]
Radiolabeled somatostatin receptor for treatment of GEP-NETs
GEP-NETs are usually slow growing. When metastasized, treatment with somatostatin analogs results in reduced hormonal overproduction and symptomatic relief in most cases. However, treatment with somatostatin analogs is seldom successful in terms of tumor size reduction.
A new treatment modality for inoperable or metastasized GEP-NETs is the use of radiolabeled somatostatin analogs. The majority of GEP-NETs posses somatostatin receptors and can, therefore, be visualized using the radiolabeled somatostatin analog. A logical sequence to this tumor visualization in patients was to treat these patients with radiolabeled somatostatin analogs. Initial studies with high dosages of [111In-DTPA0] octreotide in patients with metastatized NET are encouraging, although partial remissions (PRs) were exceptional. This is not surprising because 111In-coupled peptides are not ideal for peptide receptor radionuclide radiotherapy (PRRT) because of the small particle range and, therefore, short tissue penetration of the Auger electrons.[40,41,42,43,44]
Another radiolabeled somatostatin analog that is used for PRRT is 90Y-labeled peptides. The high energy emitting _-emitting, 90Y (Eave, _=0.935 MeV) with its longer range in tissue (Rmax = 11.3 mm), appears more suitable for therapeutic applications.[45] This is particularly true for the treatment of larger solid tumors taking into consideration the expected non-homogeneous distribution receptor expression. Therefore, the increased probability of cell killing is attributed to the cross-fire effect. However, there is a relatively high radiation exposure to normal tissue, such as liver, kidneys, and spleen.[46]
90Y-DOTATOC, 90Y-DOTATATE, and 90Y-lanreotide are the principal 90Y-labeled radiopharmaceuticals used for PRRT therapy. As 90Y is a pure beta-emitter, it is fairly difficult to conduct post-treatment, patient specific dosimetry to estimate the tumor and organ specific irradiation doses. Alternative methods were therefore suggested e.g. applying simultaneous or pre-treatment imaging studies using the same analogue labeled with 111In or substituting 90Y by the positron emitter86Y.[47,48,49,50,51]
Several groups have been performed using this compound. Complete remission (CR) and partial remission (PR) has been reported in 7% to 33% of patients with GEPNETs. [52,53,54,55,56]
The predicted absorbed doses of critical organs were shown a wide range that were (range, 1.06-10.3 mGy/MBq) for kidneys, (range, 0.1-2.6 mGy/MBq) for liver and (range, 1.5-19.4 mGy/MBq) for spleen.[57,58] The major drawback of 90Y-peptides is that activity administered is limited by the high renal dose, which can preclude the achievement of a prescribed tumor dose.
The somatostatin analogs [DOTA0, Tyr3] octreotate has a nine-fold higher affinity for the somatostatin receptor subtype2 compared with [DOTA0, Tyr3] octreotide in vitro. Also labeled with the beta- and gamma-emitting radionuclide 177Lu, this compound was shown to be successful in terms of tumor regression and animal survival in a rat model.
The use of two radionuclides delivered unwanted radiation and made the things very complex which required expert worker as used with 90Y-labeled peptide therapy. 177Lu labeled with peptides came in practice for treatment of neuroendocrine tumors from last decade and is considered as the most effective radionuclide.
177Lu is _-emitter (Emax=0.50 MeV), with a long half-life (6.73 d). It is also a ã-emitter of low-emission abundance (113[6%] and 208 [11%] keV). These characteristics enable imaging and therapy with the same complex and allow dosimetry to be performed before and during treatment as well. Comparison of its penetration range in tissue (Rmax = 2 mm) with 90Y indicates a lower cross-fire effect partially compensated by a higher percentage of the radiation energy absorbed in very small volumes. This makes 177Lu a good candidate nuclide for the treatment of small tumors (<2 cm) and micro-metastases with 177Lu-DOTATATE.[59,60]
Kwekkeboom et al compared the [177Lu-DOTAO-Tyr3] Octreotide with [111In-DTPA0] Octreotide in patients with neuroendocrine tumors and found similar biologic half lives for both radionuclides, with providing higher absorbed dose to most tumors by 177Lu.[61]
The mean absorbed dose to kidneys, spleen and tumor from 177Lu-DOTATATE and 177Lu-DOTANOC were shown in Table 3.[62]
The blood clearance and urinary excretion are fast. Dosimetric data on 177Lu-DOTATATE and 177Lu-DOTANOC are still limited because of limited available of literature related to these radiopharmaceuticals. 177Lu-DOTATATE delivered high radiation to tumors with low radiation to normal organ as compared to 177Lu-DOTATANOC and 90YDOTATOC. Dosimetry of 177Lu-DOTATAE with ranges of 1.8- 2.7mGy/MBq to spleen, 0.3-1.0 mGy/MBq to the liver, and1.0- 2.2 mGy/MBq to the kidneys. Table 4 shows clinical efficacy of radiolabled somatostatin analogs for the treatment of NETs. This therapy is well established in Europe (Germany and Netherland).
Post therapy Images of 177Lu-DOTATATE of patient with NETs confirmed from 68Ga-DOTATOC PET/CT scan (of same patient shown in Figure1) are shown in Figure 2. These images are acquired with Mill VG Dual Head Gamma Camera.
Future radionuclide and peptides for PRRT
Some radionuclides investigated in preclinical studies have given valuable results for radionuclide therapy. 166Ho, 188Re, 64Cu and 67Cu are important future radionuclides for treatment of neuroendocrine tumors. Bombesin derivatives with 166Ho and 188 Re and bombesin and somatostatin derivatives like 64Cu-DOTA-PEG-BN are labeled. 64Cu-TETAoctreotide showed clear lesion detection and had favorable pharmacokinetics.[63]
Cu-labeled peptides both 64 Cu and 67 Cu are suitable for imaging and therapy and they might delivered optimum radiation absorbed dose to tumors which data are limited.[64,65,66,67,68,69] Other radionuclides like 211Bi and 225At (a-emitter radionuclides) could be labeled with peptides and are under labeling and pre-clinical stage.
Safety aspects and radiation protection of kidneys in PRRT
Even though the radiolabeled peptides show fast clearance from blood, they are localized in liver, spleen and kidneys due to normal physiology of the body. Since no spleen toxicity has ever been found after the therapy, it was clear from beginning that the kidneys are the critical organs, owing to the renal interstitial irradiation derived from tubular peptides re-absorption as radiolabeled peptides are mainly excreted through kidneys. The cumulative absorbed dose to the kidneys may cause renal damage above the conventional threshold dose of 23Gy [according to National Council on Radiation Protection and Measurements (NCRPM) a dose of 23Gy to the kidneys caused detrimental deterministic effects in 1-5% of patients.[70]
In our opinion, kidneys must be considered the doselimiting organ in receptor radionuclide therapy. As the 23Gy limits were derived from external radiotherapy, doubts were raised about the real threshold dose for kidney toxicity with internal emitters: it was suggested that this might be higher due to the different kinetics of irradiation exposure, which typically decreases with time. There is still much to learn about renal toxicity and its avoidance. Nevertheless, caution is justified by the necessity to avoid renal failure, as reported in the literature.
Since, the high kidney uptake of radiolabeled peptide and the radio- sensitivity of renal parenchyma, appropriate methods of reducing kidney uptake appear fully justified in order to avoid acute or delayed renal toxicity. Sporadic cases of delayed renal failure have indeed been observed, especially in patients who have received > 7.4 GBq/m2. [71,72,73] Various methods like saline infusion on renal radioactivity and infusion of different amino acid solutions on renal radioactivity have been used to protect the kidneys from radiation.
Recent reports show that cationic amino acids, like lysine and arginine, interfere with proximal tubule cell protein reabsorption system in general[74] and with the uptake of radiolabeled peptides.[75]The mechanism of the inhibition by these positive charged amino acids may be competition for negative charged on the tubule cell membrane between the amino acid and radiolabeled peptides.Hammond et al reported inhibition of renal uptake of 111In-DTPA-octreotide in patients with commercially viable mixture of amino acid containing, among others, 2.46 g of lysine and 8.9 g of arginine.[76]
Currently used mixtures of amino acids do have several disadvantages, namely the induction of severe vomiting, the risk of metabolic changes like serious hyperkalaemia and the large volume needed because of the hyperosmolarity of the fluid. Furthermore, administration of cationic amino acids like arginine and lysine has been associated with serious hyperkalaemia 77, as well as having the potential to cause fatal arrhythmias. Barone et al78 also studied different amino acid solutions. Their data showed that with infusion of their most effective amino acid solution, 40% higher doses of 86YDOTATOC could be administered without increasing the renal radiation dose. Rolleman et al79 tested various amino acid solutions in patients receiving PRRT with radiolabeled Octreotide in order to assess their capacity to inhibit the renal uptake of radioactivity and their safety. Seventy-five grams of lysine resulted in the best inhibition of renal radioactivity, but produced unacceptably severe hyperkalaemia. It is therefore not a suitable regimen for clinical use. The combination of LysArg yielded the second best results with regard to inhibition of renal radioactivity but was far safer: no serious hyperkalaemia was found and no vomiting occurred. Thus, inhibition of renal radioactivity, which is dose limiting for PRRT, with the combination of 25 g of lysine and 25 g of arginine (LysArg) is effective and safe.
Conclusion
Radiolabeled somatostatin analogs are new radioligands that are used for staging of (recurrence of) NETs. The future application of radiolabeled somatostatin analogs in tumor scintigraphy may be aimed at their in vivo use as prognostic predictors and not necessarily for localization per se. SRS is readily available and assists in decisions about treatment with somatostatin analogs, either unlabeled or radiolabeled. Therefore, SRS still is an important radionuclide imaging method in NETs. PET-CT with either 68Ga-DOTATOC or 18FFDOPA, however, is more accurate than conventional radionuclide imaging using SRS. PRRT has proven to be a safe and effective treatment for neuroendocrine tumors. Present knowledge and experience indicates that it is possible to deliver high activities, and therefore high absorbed doses, to tumors expressing SST2 receptors, with achievement of objective therapeutics responses. Patient variability requires careful individual dosimetry to determine the activity to be administered in PRRT. Radiation protection of kidneys could be minimized by infusion of positive amino acid (Lys-Agr). However, the value of the kidney absorbed dose may not be sufficient to accurately predict the likelihood of renal toxicity for individual patients. Other factors contribute to the biologic effectiveness, including the tissue radiosensitivity, dose rate and cycle therapy scheme. Radiosensitizers could be used to increase the radiosensitivity of tumors for better treatment as these methods are common in practice in external beam radiotherapy.
Acknowledgements
We greatly appreciate and acknowledge the invaluable contributions of Dr Meera Venkatesh, Dr Sharmila Banerjee, Dr Tapas Das and Dr Sudipto Chakraborty of BARC and Dr MRA Pillai of IAEA to start 177Lu- DOTATATE therapy at AIIMS.
References
1. Krulich L, Dhariwal AP, and McCann SM. Stimulatory and inhibitory effects of purified hypothalamic extracts on growth hormone release from rat pituitary in vitro. Endocrinology. 1968;83:783–90.
2. Brazeau P, Vale W, Burgus R, Ling N, Butcher M, Rivier J, et al. Hypothalamic polypeptide that inhibits the selection of immunoreactive pituitary growth hormone. Science 1973,179:77–9
3. Hellman B, Lernmark A. Inhibition of the in vitro secretion of insulin by an extract of pancreatic alpha-1 cells. Endocrinology. 1969;84:1484–8.
4. Reichlin S. Somatostatin. N Engl J Med. 1983;309:1495–501.
5. Reichlin S. Somatostatin. N Engl J Med. 1983;309:1556–63.
6. Shulkes A. Somatostatin: Physiology and clinical applications. Bailliere´s Clin Endocrinol Metab. 1994;8:215–36.
7. Kwekkeboom D, Krenning EP, de Jong M. Peptide Receptor Imaging and Therapy. 2000;41:1704–13.
8. Reubi JC, Schaer JC, Markwalder R, Waser B, Horisberger U, Laissue J. Distribution of somatostatin receptors in normal and neoplastic human tissues: recent advances and potential relevance. Yale J Biol Med. 1997;70:471–9.
9. Machac J, Krynyckyi B, Kim C. Peptide and Antibody Imaging in lung Cancer. Semin Nucl Med. 2002;32:276–92.
10. Reubi JC, Waser B. Concomitant expression of several peptide receptors in neuroendocrine tumors: molecular basis for in vivo multireceptor tumor targeting. Eur J Nucl Med Mol Imaging. 2003;30:781–93.
11. Warner RR. Enteroendocrine tumors other than carcinoid: a review of clinically significant advances. Gastroenterology. 2005;128:1668–84.
12. Modlin IM, Oberg K, Chung DC, Jensen RT, de Herder WW, Thakker RV, et al. Gastroenteropancreatic neuroendocrine tumours. Lancet Oncol. 2008;9:61–72.
13. Modlin IM, Lye KD, Kidd M. A 5–decade analysis of 13,715 carcinoid tumors. Cancer. 2003;97:934–59.
14. Hemminki K, Li X. Incidence trends and risk factors of carcinoid tumors: a nationwide epidemiologic study from Sweden. Cancer. 2001;92:2204–10.
15. Levi F, Te VC, Randimbison L, Rindi G, La Vecchia C. Epidemiology of carcinoid neoplasms in Vaud, Switzerland, 1974-97. Br J Cancer. 2000;83:952–5.
16. Ramage JK, Davies AH, Ardill J, Bax N, Caplin M, Grossman A, et al. Guidelines for the management of gastroenteropancreatic neuroendocrine (including carcinoid) tumours. Gut. 2005;54:iv1–16
17. Rehfeld JF. The new biology of gastrointestinal hormones. Physiol Rev. 1998;78:1087–108.
18. Alexakis N, Neoptolemos JP. Pancreatic neuroendocrine tumours. Best Pract Res Clin Gastroenterol. 2008;22:183–205.
19. Duerr EM, Chung DC. Molecular genetics of neuroendocrine tumors. Best Pract Res Clin Endocrinol Metab. 2007;21:1–14.
20. Toumpanakis CG, Caplin ME. Molecular genetics of gastroenteropancreatic neuroendocrine tumors. Am J Gastroenterol. 2008;103:729–32.
21. Oberg K . Neuroendocrine tumors of the gastrointestinal tract: recent advances in molecular genetics, diagnosis, and treatment . Curr Opin Oncol. 2005;17:386–91.
22. Massironi S, Sciola V, Peracchi M, Ciafardini C, Spampatti MP, Conte D. Neuroendocrine tumors of the gastro-enteropancreatic system. Worl J Gastroenterol. 2008;14:5377–84.
23. Tamm EP, Kim EE, Ng CS. Imaging of neuroendocrine tumors. Hematol Oncol Clin North Am. 2007;21:409–32.
24. Becherer A, Szabó M, Karanikas G, Wunderbaldinger P, Angelberger P, Raderer M, et al. Imaging of advanced neuroendocrine tumors with (18)F-FDOPA PET. J Nucl Med. 2004;45:1161–7.
25. Intenzo CM, Jabbour S, Lin HC, Miller JL, Kim SM, Capuzzi DM, et al. Scintigraphic imaging of body neuroendocrine tumors. Radiographics. 2007;27:1355–69.
26. Krenning EP, Kwekkeboom DJ, Oei HY, de Jong RJ, Dop FJ, de Herder WW, et al. Somatostatin receptor scintigraphy in carcinoids, gastrinomas and Cushing’s syndrome. Digestion. 1994;55:54–9.
27. Kubo H, Chijiiwa Y, Akahoshi K, Hamada S, Matsui N, Nawata H, et al. Pre-operative staging of ampullary tumors by endoscopic ultrasound. Br J Radiol. 1999;72:443–7.
28. Kwekkeboom DJ, Krenning EP. Somatostatin receptor imaging. Semin Nucl Med. 2002;32:84–91.
29. Reed NS. Management of neuroendocrine tumours. Clin Oncol (R Coll Radiol). 1999;11:295–302.
30. Eriksson B, Bergstrom M, Orlefors H, Sundin A, Oberg K, Långström B. Use of PET in neuroendocrine tumors. In vivo applications and in vitro studies. Q J Nucl Med. 2000;44:68–76.
31. Henze M, Schuhmacher J, Hipp P, Kowalski J, Becker DW, Doll J, et al. PET Imaging of somatostatin receptors using[68GA]DOTAD- Phe1-Tyr3-Octreotide: first results in patients with meningiomas. J Nucl Med. 2001;42:1053–6.
32. As
33. S
34. Maecke HR, Hofmann M,and Haberkorn U: (68)Ga-labeled peptides in tumor imaging. J Nucl Med. 2005;46:172S–8S.
35. Baum RP, Schmucking M, Niesen A, et al: Receptor-PET/CT of neuroendocrine tumors using the gallium-68 labelled somatostatin analogs DOTA-NOC: first clinical results. Euro Radiol. 2005;1S:C-0409A.
36. R.P. Baum and D. Horsch. Efficient Diagnosis of Neuroendocrine Tumors of the Gastro-entero-pancreatic System.
37. Wängberg B, Nilsson O, Johanson V V, Kölby L, Forssell- Aronsson E, Andersson P, et al. Somatostatin Receptors in the Diagnosis and Therapy of Neuroendocrine Tumor. Oncologist. 1997;2:50–8.
38. Becherer A, Szabó M, Karanikas G, Wunderbaldinger P, Angelberger P, Raderer M, et al. Imaging of advanced neuroendocrine tumors with 18F-FDOPA PET. J Nucl Med. 2004;45:1161–67.
39. Siegel JA, Thomas SR, Stubbs JB, Stabin MG, Hays MT, Koral KF, et al. MIRD Pamphlet No.16: Techniques for quantitative radiopharmaceutical biodistribution data acquisition and analysis for use in human radiation dose estimates. J Nucl Med. 1999;40:37S–61S.
40. Stabin MG, Kooij PP, Bakker WH, Inoue T, Endo K, Coveney J, et al. Radiation dosimetry for indium-111- pentetreotide. J Nucl Med. 1997;38:1919–22.
41. Capello A, Krenning EP, Breeman WA, Bernard BF, de Jong M, et al. Peptide receptor radionuclide therapy in vitro using [111In- DTPA0]octreotide. J Nucl Med. 2003;44:98–104.
42. Valkema R, De Jong M, Bakker WH, Breeman WA, Kooij PP, Lugtenburg PJ, et al. Phase I study of peptide receptor radionuclide therapy with [In-DTPA]octreotide: the Rotterdam experience. Semin Nucl Med. 2002;32:110–22.
43. Forrer F, Uusijarvi H, Waldherr C, Cremonesi M, Bernhardt P, Mueller-Brand J, et al. A comparison of (111)In-DOTATOC and (111)In-DOTATATE: biodistribution and dosimetry in the same patients with metastatic neuroendocrine tumours. Eur J Nucl Med Mol Imaging. 2004;31:1257–62.
44. Cremonesi M, Ferrari M, Bodei L, Tosi G, Paganelli G. Dosimetry in Peptide radionuclide receptor therapy: a review. J Nucl Med. 2006;47:1467–75.
45. Heppeler A, Froidevaux S,Eberle AN, Maecke HR. Receptor targeting for tumor localization and therapy with radiopeptides. Curr Med Chem. 2000;7:971–94.
46. Harrison A, Walker CA, Parker D, Jankowski KJ, Cox JP, Craig AS, et al. The in vivo release of 90Y from cyclic and acyclic ligand-antibody conjugates. Int J Rad Appl Instrum B. 1991;18:469–76.
47. Forster GJ, Engelbach MJ, Brockmann JJ, Reber HJ, Buchholz HG, Mäcke HR, et al. Preliminary data on biodistribution and dosimetry for therapy planning of somatostatin receptor positive tumors: comparison of 86(Y)-DOTATOC and (111)In-DTPAoctreotide. Eur J Nucl Med. 2001;28:1743–50.
48. Helisch A, Forster GJ, Reber H, Buchholz HG, Arnold R, Göke B, et al. Pre-therapeutic dosimetry and biodistribution of 86Y-DOTAPhe 1-Tyr 3-octreotide versus 111In-pentetreotide in patients with advanced neuroendocrine tumours. Eur J Nucl Med Mol Imaging. 2004;31:1386–92.
49. Cremonesi M, Ferrari M, Zoboli S, et al. Biokinetics and dosimetry in patients administered with (111)In-DOTA-Tyr(3)-octreotide: implications for internal radiotherapy with (90)Y-DOTATOC. Eur J Nucl Med. 1999;26:877–86.
50. No
51. De Jong M, Bakker WH, Breeman WA, Bernard BF, Hofland LJ, Visser TJ, et al: Pre-clinical comparison of [DTPA0] octreotide, [DTPA0,Tyr3] octreotide and [DOTA0,Tyr3] octreotide as carriers for somatostatin receptor-targeted scintigraphy and radionuclide therapy. Int J Cancer. 1998;75:406–11.
52. Valkema R, Jamar F, Jonard P et al: Targeted radiotherapy with 90Y-DOTA-Tyr3-octreotide (90YSMT487; OctreoTherTM): a phase I study. J Nucl Med (abstract). 2000;41.(suppl):111P.
53. Buscombe JR, Caplin ME, Hilson AJ. Long-term efficacy of highactivity 111in-pentetreotide therapy in patients with disseminated neuroendocrine tumors. J Nucl Med. 2003;44:1–6.
54. Paganelli G, Zoboli S, Cremonesi M, Macke HR, Chinol M. Receptormediated radionuclide therapy with 90Y-DOTA-D-Phe1-Tyr3- Octreotide: preliminary report in cancer patients. Cancer Biother Radiopharm. 1999;14:477–83.
55. Waldherr C, Pless M, Maecke HR, Haldemann A, Mueller-Brand J. The clinical value of [90Y-DOTA]-D-Phe1-Tyr3- octreotide (90YDOTATOC) in the treatment of neuroendocrine tumours: a clinical phase II study. Ann Oncol. 2001;12:941–5.
56. Virgolini I, Britton K, Buscombe J, Moncayo R, Paganelli G, Riva P. In- and Y-DOTA-lanreotide: results and implications of the MAURITIUS trial. Semin Nucl Med. 2002;32:148–55.
57. Forrer F, Uusijarvi H, Waldherr C, Cremonesi M, Bernhardt P, Mueller-Brand J, et al. A comparison of (111)In-DOTATOC and (111)In-DOTATATE: biodistribution and dosimetry in the same patients with metastatic neuroendocrine tumours. Eur J Nucl Med Mol Imaging. 2004;31:1257–62.
58. Cremonesi M, Ferrari M, Zoboli S, Chinol M, Stabin M, Orsi F, et al Biokinetics and dosimetry in patients administered with (111)In- DOTA-Tyr(3)-octreotide: implications for internal radiotherapy with 90Y-DOTATOC. Eur J Nucl Med. 1999;26:877–86.
59. Kassis AI, Adelstein SJ. Radiobiologic Principles in Radionuclide therapy. J Nucl Med. 2005;46 :4S–12.
60. Siegel JA,Stabin MG. Absorbed fractions for electrons and beta particles in spheres of various sizes. J Nucl Med. 1994;35:152–6.
61. Kwekkeboom DJ, Bakker WH, Kam BL, Teunissen JJ, Kooij PP, de Herder WW, et al. Treatment of patients with gastro-enteropancreatic (GEP) tumours with the novel radiolabelled somatostatin analogue [177Lu-DOTA(0),Tyr3]octreotate. Eur J Nucl Med Mol Imaging. 2003;30:417–22.
62. Wehrmann C, Senftleben S, Zachert C, Müller D, Baum RP. Results of individual patient dosimetry in peptide receptor radionuclide therapy with 177Lu DOTA-TATE and 177Lu DOTA-NOC. Cancer Biother Radiopharm. 2007;22:406–16.
63. Kaltsas GA, Papadogias D, Makras P, Grossman AB. Treatment of advanced neuroendocrine tumours with radiolabelled somatostatin analogues. Endocr Relat Cancer. 2005;12:683–99.
64. Wester HJ, Schottelius M, Scheidhauer K, Meisetschläger G, Herz M, Rau FC, et al. PET imaging of somatostatin receptors: design, synthesis and preclinical evaluation of a novel 18F-labelled, carbohydrated analogue of octreotide. Eur J Nucl Med Mol Imaging. 2003;30:117–22.
65. Kaltsas GA, Papadogias D, Makras P, Grossman AB. Treatment of advanced neuroendocrine tumours with radiolabelled somatostatin analogues. Endocr Related Cancer. 2005;12:683–99.
66. Wester HJ, Schottelius M, Scheidhauer K, et al: PET imaging of somatostatin receptors: design, synthesis and preclinical evaluation of a novel 18F-labelled, carbohydrated analogue of octreotide. Eur J Nucl Med Mol Imag. 2003;30:117–122
67. Chen X, Park R, Hou Y, Tohme M, Shahinian AH, Bading JR, et al MicroPET and autoradiographic imaging of GRP receptor expression with 64Cu-DOTA-[Lys3]bombesin in human prostate adenocarcinoma xenografts. J Nucl Med. 2004;45:1390–7.
68. Rogers BE, Manna DD, Safavy A. In vitro and in vivo evaluation of a 64Cu-labeled polyethylene glycol-bombesin conjugate. Cancer Biother Radiopharm. 2004;19:25–34.
69. Anderson CJ, Dehdashti F, Cutler PD, Schwarz SW, Laforest R, Bass LA, et al. 64Cu-TETA-octreotide as a PET imaging agent for patients with neuroendocrine tumors. J Nucl Med. 2001;42:213–21.
70. National Council on Radiation Protection and Measurements (NCRPM). Misadministration of Radioactive Material in Medicine- Scientific Background. Bethesda MD: NRCPM, 1991:27.
71. Behr TM, Behe M, Kluge G, Gotthardt M, Schipper ML, Gratz S, et al. Nephrotoxicity vs anti-tumor efficacy in radiopeptide therapy: facts and myths about the Scylla and Charybdis. Eur J Nucl Med Mol Imaging. 2002;29:277–9.
72. Cybulla M, Weiner SM, Otte A. End-stage renal disease after treatment with 90Y-DOTATOC. Eur J Nucl Med. 2001;28:1552–4.
73. Otte A, Herrmann R, Heppeler A, Behe M, Jermann E, Powell P, et al. Yttrium-90 DOTATOC: first clinical results. Eur J Nucl Med. 1999;26:1439–47.
74. Mogensen CE, Solling. Studies on renal tubular protein reabsorption: partial and near complete inhibition by certain amino acids. Scand J Clin Lab Invest. 1977;37:477–86.
75. Behr TM, Goldenberg DM, Becker W. Reducing the renal uptake of radiolabeled antibody fragments and peptides for diagnosis and therapy; present status, future prospects and limitations. Eur J Nucl Med. 1998;25:201–12.
76. Hammond PJ, Wade AF, Gwilliam ME, Peters AM, Myers MJ, Gilbey SG, et al. Amino acid infusion blocks renal tubular uptake of an indium-labeled somatostatin analogue. Br J Cancer. 1993;67:1437–9.
77. Massara F, Cagliero E, Bisbocci D, Passarino G, Carta Q, Molinatti GM. The risk of pronounced hyperkalaemia after arginine infusion in the diabetic subject. Diabete Metab. 1981;7:149–53.
78. Barone R, De Camps J, Smith C, et al. Metabolic effect of amino acids (AA) solutions infused for renal radioprotection. J Nucl Med (abstract). 2000;41:94P
79. Rolleman EJ, Valkema R, de Jong M, Kooij PP, Krenning EP. Safe and effective inhibition of renal uptake of radiolabelled octreotide by a combination of lsine and arginine. Eur J Nucl Med Mol Imaging. 2003;30:9–15.