BS Ramakrishna
Department of Gastrointestinal Sciences
Christian Medical College
Vellore 632004, India.
Corresponding Author:
Dr. BS Ramakrishna
Email: rama@cmcvellore.ac.in
Abstract
There is resurgent interest in the use of probiotics to maintain gastrointestinal and systemic health, driven by recent advances in knowledge of bacterial interactions with the epithelium and innate immune system of the intestine. The effects of probiotic bacteria on the intestinal epithelium and their downstream consequences are reviewed. Probiotics prevent pathogen adherence and invasion of the epithelium, partly by blocking adherence sites but also by upregulating gene expression of MUC2 and of antimicrobial peptides. Metabolic effects of probiotics on the intestinal epithelium include production of short chain fatty acids which influence epithelial cell metabolism, turnover and apoptosis. Bacterial metabolism of unabsorbed dietary constituents with production of free radicals and phenolic metabolites can lead to DNA damage and cancer; probiotics restore eubiosis and potentially prevent this. Probiotics alter expression and redistribution of tight junction proteins and reduce intestinal permeability limiting absorption of noxious molecules from the gut lumen. Most studied are the effects of probiotics on epithelial cells which are the first line of innate immune-capable cells that encounter luminal flora. Probiotics, through secreted molecules, influence the innate inflammatory response of epithelial cells to stimuli from the gut lumen, and reduce mucosal inflammation. Through effects on dendritic, and possibly epithelial, cells they influence naïve T cells in the lamina propria of the gut and thus influence adaptive immunity. These varied effects of probiotics have implications for the treatment of several gastrointestinal diseases including antibiotic-associated colitis, acute gastroenteritis, inflammatory bowel disease, colon cancer, and irritable bowel syndrome.
|
48uep6bbphidvals|200 48uep6bbphidcol2|ID 48uep6bbph|2000F98CTab_Articles|Fulltext Probiotics (probiosis = for life) are defined as living microorganisms which, when administered in adequate amounts, confer health benefits on the host.[1,2] Although fermented foods containing live micro-organisms have been used for centuries, the recent surge of interest in probiotic preparations is attributed to our increased understanding of the innate immune system and how it distinguishes and reacts to harmless or beneficial bacteria as opposed to harmful bacteria. Probiotics are ingested; they survive gastric acid and duodenal bile and reach the small and large bowels to exert their effects. Their first prolonged contact with the human host is with the epithelium of the gastrointestinal tract, which thus acquires much significance when trying to unravel the physiological effects of probiotics.
Probiotics must be ingested regularly for any health promoting activity to persist. It is possible to manipulate (at least temporarily) the composition of the intestinal microflora through dietary supplementation with probiotics. The wide marketing of probiotics throughout the world confirms the popularity of this concept. Most probiotics in current use comprise bacteria, either Lactobacilli or Bifidobacteria, although some yeast species are also used. Lactobacilli are Gram-positive, non-spore forming rods or coccobacilli.3 They are found in a variety of habitats where rich, carbohydratecontaining substrates are available, such as human and animal mucosal membranes, on plants or material of plant origin, sewage and fermenting or spoilt food. Lactobacilli are normally found in the intestine of infants in high numbers, but decline rapidly after infancy.[4] Bifidobacteria are non-motile, non-sporulating Gram-positive rods with varying appearance. Most strains are strictly anaerobic. They constitute a major part of the normal intestinal microflora in humans,[5] appearing in the stools a few days after birth and increasing in number thereafter. The number of bifidobacteria in the colon of young children is high, but this number decreases rapidly with age.[4]
Probiotics may influence gastrointestinal health in a variety of ways (Table I). This review outlines the scientific evidence which suggests that interactions of probiotic microorganisms with the intestinal epithelium leads to health benefits in the gastrointestinal tract.
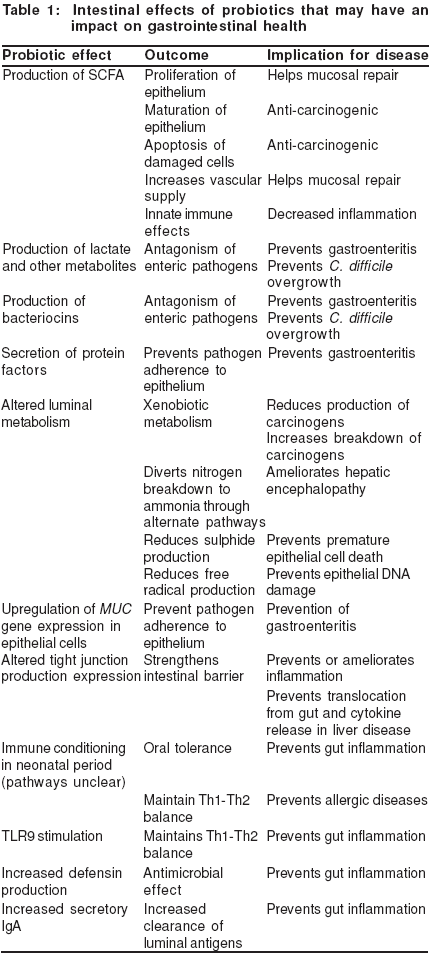
Probiotics and effects on intestinal luminal metabolism and epithelial cell function
The human gastrointestinal tract contains over 500 species of bacteria. Some of these are harmless to the host, some are beneficial and yet others are harmful to the host. In health there is an optimum gut flora balance; the beneficial bacteria, such as Lactobacilli and Bifidobacteria, predominate. It is suggested that the balance should be such that at least 85% of the intestinal microflora in a healthy person should be beneficial bacteria.6 Under certain conditions this balance, or eubiosis as it has been eloquently termed, is lost leading to dysbiosis. A reduction in beneficial bacteria such as Lactobacilli and Faecalibacterium prausnitzii have been implicated in the pathogenesis of inflammatory bowel disease and to a lesser extent in colon cancer.[7,8,9,10,11] Such an altered bacterial balance may potentially change fluxes through the metabolic pathways in epithelial cells, or it may alter immune balance through altered innate immune activation involving epithelial and subepithelial cells. The regular ingestion of probiotics is one possible way of restoring balance in the intestinal microbial ecosystem.
Luminal antagonism of enteric pathogens
Probiotics can provide protection against a broad range of pathogens, including certain forms of Clostridia, Escherichia coli, Salmonella, Shigella, Pseudomonas, and yeasts such as Candida albicans. They may inhibit the growth of intestinal pathogens by competing for nutrients consumed by pathogenic bacteria or by producing substances that directly affect growth of pathogens. These inhibitory substances include metabolites such as lactic acid, short chain fatty acids and hydrogen peroxide, that are inhibitory to both gram-positive and gram-negative bacteria.[12] They also include bacteriocins and other soluble factors that inhibit the growth of pathogenic bacteria. Lactobacilli produce bacteriocins such as sakacin, lactocin, amylovorin and acidophilin.[13] They exert antimicrobial activity in vitro against pathogenic E. coli, Salmonella, Bacillus cereus, Campylobacter jejuni and H. pylori. Bifidobacteria are also known to produce bacteriocins (eg. bifidin and bifidocin) and have antimicrobial activity against several pathogens including E. coli, B. cereus, and S. aureus.[13,14,15]
Blockage of epithelial adherence and invasion by pathogens
Probiotic Lactobacilli and Bifidobacteria can adhere to intestinal epithelial cells through surface-expressed proteins.[16,17] In the case of Lactobacillus casei it has been shown that the bacteria bind to extracellular matrix components such as collagen, fibronectin or fibrinogen.[18] It is hypothesized that some secreted factors of probiotics may inhibit binding of pathogenic bacteria to the appropriate receptor on the epithelial surface in addition to inhibiting epithelial cell invasion by the pathogen.[ 16,17,19,20,21] The surface of the intestine is covered by mucus, which contains mucins secreted by intestinal epithelial cells as a major component. It has been shown that probiotic Lactobacilli upregulate the MUC2 mucin protein in epithelial cell lines[22,23] and inhibit attachment of enterohemorrhagic E. coli.[23] These findings have correspondingly also been detected in vivo in rats, where a probiotic mixture of Lactobacilli and bifidobacteria increased the secretion of mucin and stimulated MUC2 gene expression (with minor effects on MUC1 and MUC3 gene expression) in the colon.[24] The Lactobacilli present in the mixture were the most potent in stimulating colonic mucin secretion. A major stimulatory effect of Lactobacilli on MUC3 mucin mRNA expression and extracellular MUC3 secretion has been shown in the HT29 colonic epithelial cell line.[25]
Effects of probiotics on luminal metabolism
Bacteria produce a variety of metabolites, some of which are useful and others which are harmful to the human host. The colonic bacteria derive metabolic energy from unabsorbed carbohydrates in the lumen of the gut, fermenting them to various products the most important of which are short chain fatty acids. Short chain fatty acids such as acetate and butyrate influence colonic epithelial function in many ways; they are the major metabolic fuel for the epithelial cells, they influence gene expression in the epithelium, and they influence epithelial proliferation and barrier function.[26,27,28,29] Reduction in short chain fatty acid production may play a role in the pathogenesis of a variety of gastrointestinal illnesses including antibioticassociated colitis, inflammatory bowel disease, colon cancer and hepatic encephalopathy.[26] Eubacterium rectale and Faecalibacterium prausnitzii, two of the major short chain fatty acid-producing bacteria in the colon, are reduced in number in patients with colon cancer.[22] Short chain fatty acids help in differentiation of the colonic epithelium as well as in inducing apoptosis in damaged epithelial cells, an action that is likely to be useful in prevention of colon cancer. Probiotics stimulate the production of short chain fatty acids by bacteria in the colon,[30] and may potentially be useful in disease conditions where deficiency of short chain fatty acids are postulated to be involved in pathogenesis.
Bacteria in the lumen of the intestine also produce molecules that may be toxic to the intestinal epithelium. For instance bacterial metabolites, such as hydrogen sulphide produced by sulphate-reducing bacteria and extracellular superoxide produced by bacteria such as Enterococcus faecalis, can damage DNA in the colonic epithelium.[31,32,33] These changes may increase the rate of occurrence of somatic mutations, and may result in development of colon cancer in predisposed individuals. Sulphide interferes with metabolism of short chain fatty acids and thus may cause premature death of epithelial cells.[34] Epithelial cells in the colon are important sites of detoxification of bacterial metabolites, especially phenolic metabolites resulting from protein breakdown, produced in the lumen of the colon.[35,36] Alterations in the balance of bacteria in the colon may lead to the generation of more metabolites than the epithelium can cope with. For instance, damage of DNA induced by bacterial superoxide may result in somatic mutations in epithelial cells that predispose to the development of colon cancer. The administration of probiotics can potentially reduce the levels of sulphide and superoxide produced by luminal bacteria and protect epithelial cell metabolic and repair processes.
Hepatic encephalopathy is another condition in which bacterial production of ammonia and other metabolites from nitrogeneous substrates in the intestinal lumen contributes to pathogenesis. Traditionally the treatment involves administration of an antibiotic or a prebiotic (eg. Lactulose) to alter bacterial metabolism. There is now experimental evidence that luminal bacterial metabolism may be directed away from ammonia into other pathways, leading to reduced systemic blood levels of ammonia and related metabolites.[37,38]
Effects of probiotics on intestinal epithelial tight junctions
The intestinal mucosal barrier is composed of epithelial cells, the tight junctions between the cells, and the mucus layer overlying the epithelium. The tight junctions between epithelial cells are a significant component of the intestinal barrier. They are made up of a number of proteins including the claudins, occludin, zona occludens (ZO) proteins, and junctional adhesion molecules, which together determine tight junction structure and function. Tight junction structure and function is affected by a number of nutrients and bacterial molecules which affect the expression of the tight junction proteins. Tight junction protein expression may also be influenced by genetic factors, resulting in barrier defects in celiac disease and ulcerative colitis. A variety of probiotic bacteria, including Lactobacillus rhamnosus GG, Bifidobacterium infantis, Bifidobacterium lactis and Escherichia coli Nissle 1917, appear to increase tight junction integrity and prevent tight junction disruption by noxious chemical influences or secondary to microbial pathogens.[39,40,41,42,43,44] There is evidence that the effects of probiotic bacteria are mediated by soluble peptides which are secreted into the medium. The biochemical pathways mediating the probiotic effect on tight junction function include protein kinase C and MAP kinase pathways, and involve both redistribution and altered expression of the tight junction proteins occludin, ZO-1 and ZO-2 and claudins 1, 2, 3 and 4. [39,42,43]
Probiotic effects on immune conditioning via the epithelium and sub-epithelial cells
The intestine is sterile at birth. Colonisation by bacteria begins immediately after birth and is influenced by the route of delivery, hygiene of the neonatal environment, and maternal bacterial flora in the diet. The initial exposure to intestinal bacteria is very important in immune conditioning of the host. The commensal bacteria in the gastrointestinal tract are the primary stimulus for the intestinal mucosal immune system and are necessary for normal immune development. For instance, bacteria-free animals grew to develop a Th2 predominant immune system with excessive production of IL-4.[45] In these animals intestinal exposure to the single bacterium Bacteroides fragilis returned the immune balance to normal by inducing the production of interferon-g. [45] This cytokine, which is produced by CD4+, CD8+ and natural killer cells, is essential for successful clearance of intracellular pathogens such as viruses, and for host defense against malignant transformation. Using genetically modified Bacteroides fragilis in germfree animals, it has been shown that polysaccharides produced by the bacteria were important in this neonatal immune conditioning. In the absence of the relevant polysaccharide, the animals had smaller spleens and lymphoid tissue and their immune system responded with a Th2 type of response. There is evidence from clinical studies that administration of probiotics to adults may also result in production of Th1 responses and interferon-g. For example, administration of probiotics regularly increased resistance to viral infections in several settings.[46,47,48] Whilst it is not known how these effects are mediated, increase of interferon-g is a likely mechanism, given its role in containing viral infections. The intestinal epithelium and intestinal dendritic cells are both likely to play roles in the genesis of this effect of probiotics. Another implication of the immune effects of probiotics is the possibility that altered immune conditioning in the intestine in the neonatal period may predispose individuals to develop atopic diseases in later life, by skewing their immune responses to a Th2 nature. Probiotic exposure at an early age may potentially prevent expression of such diseases.
The mucosal immune system of the gastrointestinal tract is regulated in such a manner that it causes controlled inflammation. Unlimited immune activation in response to commensal bacteria could lead to the risk of inflammation. The intestinal mucosal immune system has developed specialised regulatory, anti-inflammatory mechanisms for eliminating or tolerating non-antigenic food substances and commensal micro-organisms (oral tolerance).[49] At the same time the mucosal immune system must provide local defence mechanisms against environmental threats such as invading pathogens.
This important feature is coordinated by strongly developed innate defense mechanisms ensuring appropriate functioning of the mucosal barrier,[50] existence of unique types of lymphocytes and their products, transport of polymeric immunoglobulins through epithelial cells into secretions[51] and migration and homing of cells originating from the organized tissues in the mucosa and exocrine glands.
Oral tolerance is the phenomenon where the oral administration of an antigen (to which the body is already sensitised) produces a gradual reduction in the immune or inflammatory response to it. This phenomenon is deficient in germ-free mice due to a failure to generate suppressor cells. Oral tolerance can be induced in these animals by colonization of the bowel with Bifidobacterium infantis.[52] The supernatant of Bifidobacterium breve has been shown to induce dendritic cell activation and maturation and to prolong dendritic cell survival through a toll-like receptor 2 (TLR2)-dependent pathway.[53] This is characterised by increased production of IL-10, the regulatory cytokine, which may be responsible for amelioration of inflammation.
Effects of probiotics on innate immune and inflammatory pathways in epithelial cells
The most prominent effects of probiotics on the intestinal epithelium relate to innate immunity. The immune system has two components – the innate immune system which is conserved across species[54] and provides basic but nonspecific recognition of harmful motifs in the environment, and the adaptive immune system which recognises specific antigens and reacts to them. The gastrointestinal mucosa, through its folds, villi and microvilli, provides the largest surface area in the body, in excess of 100 m2, and contains a mucosal immune system that protects it from the external environment.
The adaptive immune system in the gut, also known as the gastrointestinal-associated lymphoid tissue, has inductive and effector sites.[49] The inductive sites include the Peyer’s patches in the small intestine, isolated lymphoid follicles scattered in the lamina propria, and mesenteric lymph nodes, which together make the gut the largest lymphoid organ in the body. The effector sites include lymphocytes in the lamina propria surrounding Peyer’s patches and lymphocytes scattered throughout the epithelium. The epithelial cells that line the gut separate the lumen from the underlying lymphoid tissue. Specialised areas of epithelium, such as the follicleassociated epithelium overlying the Peyer’s patches and lymphoid follicles that contain microfold M cells, are particularly the site of endocytosis of foreign antigens or particles. Antigens may also gain entry to the basolateral surface of intestinal epithelial cells by disrupting the tight junction structure.
The intestine is rich also in cells that represent the innate immune system.[50] The epithelial and dendritic cells in the intestinal mucosa possess pattern recognition receptors (PRR) which recognise basic microbe-associated molecular patterns that distinguish broad classes of harmful and harmless bacteria. The Toll-like receptors (TLRs) are the best known of the PRRs.[55] In addition to recognising and initiating signaling cascades for taking action against harmful bacteria, these receptors also recognise some beneficial bacteria. The innate immune cells, exemplified here by the intestinal epithelial cells, secrete effector molecules including cytokines and chemokines or microbicides, in response to microbes that are perceived as pathogenic. Cytokines or chemokines recruit inflammatory and immune cells to the intestine. They may also influence the development of adaptive immunity. Antigens, taken up by the microfold cells covering lymphoid nodules, are processed through antigen presenting cells to influence T cell differentiation into Th1 or Th2 cells, or T regulatory cells. Th1 activation is characterised by overproduction of interferon-g and tumor necrosis factor-a that, when excessive, leads to inflammation. Th2 activation on the other hand is characterised by the cytokines interleukin-4 and interleukin-5 and when excessive leads to allergic diseases.
T regulatory cells maintain the balance between Th1 and Th2 polarisation. It is now considered that probiotic bacteria, through their effect on the innate immune system, play significant ongoing roles in modulating T cell differentiation and polarisation of the immune response. The epithelial cells of the intestine participate in the innate immune responses to luminal bacteria and provide communication between luminal stimulants and underlying lymphoid elements in the lamina propria. In response to interaction with microbes in the lumen, the epithelium regulates the transcription of inflammatory cytokines for excretion into the lumen and interstitium and upregulates surface molecules, such as class-II antigens and the polymeric immunoglobulin-A (poly-IgA) receptor, for control in handling foreign antigens.[51] This interaction with luminal pathogens and commensal flora with the gut is termed microbial-epithelial ‘’cross talk’’ and represents an important contributor to intestinal barrier function.
The cytokine and chemokine response of epithelial cells has been examined by observing their interaction with bacterial pathogens. A variety of bacterial and viral pathogens upregulate the expression of chemokines in intestinal epithelial cells.[56,57,58,59,60] Most prominent among these are interleukin 8 and CXCL-5. This ability of epithelial cells to produce various factors promoting the infiltration of neutrophils and lymphocytes to the site of infection serves as an ‘early warning system’, whereby the epithelium signals to the underlying immune and inflammatory cells. Probiotics block the release of chemokines such as IL-8 and of inflammatory cytokines from epithelial cells in response to pathogenic bacteria or other inflammatory stimuli,[59,61] and diminish pathogen-induced epithelial cell death.[62] Pre-incubation with Lactobacillus casei protected intestinal epithelial cells against Shigella flexneri infection.[63] Blockade by probiotics of the pro-inflammatory response of the intestinal epithelium to both Shigella and Vibrio cholera appears to be mediated through blockage of inhibitory Kb degradation leading to suppression of NFkB signaling.[59,63] Secreted factors may also be responsible for the effect of probiotics in preventing pathogen infection of epithelial cells, and these have been shown to act through ERK and p38 pathways to reduce interleukin-8 secretion.[64] Probiotic Lactobacilli and bifidobacteria reduced IL-8 secretion and increased IL-10 secretion in response to Listeria monocytogenes in an epithelial cell line.[65] LPS-induced NFkB activation and inflammatory gene expression in HT-29 cells were attenuated by probiotic bifidobacteria.[66] B. infantis and L. salivarius attenuated IL-8 secretion at baseline and in response to S. typhimurium and flagellin.67 Upregulation of MUC2 gene expression in epithelial cell lines also constitutes a part of the defense system activation by probiotics.[68]
The anti-inflammatory effects of probiotics on the intestinal epithelium have also been shown in other experimental situations. Bifidobacteria modulated inflammatory cytokine secretion in biopsies from ulcerative colitis patients.69 Similarly, probiotic bacteria down-regulated secretion of tumour necrosis factor-a in explant organ cultures of colonic mucosa from patients with inflammatory bowel disease or animals with experimental colitis.[70,71]
On the other hand, probiotic bacteria may also induce production of pro-inflammatory cytokines by the intestinal epithelium. It has been reported that the probiotic microorganism Lactobacillus reuteri transiently induced interleukin IL-1, IL-6, interferon-gamma-inducible protein 10, and macrophage inflammatory protein 2, while transiently decreasing the regulatory proteins A20 and Toll-interacting protein, in intestinal epithelial cells in primary culture.[72] It has been hypothesised that this transient activation in the presence of established microflora may aid mucosal homeostasis.
The Paneth cells in the intestinal epithelium secrete antimicrobial peptides such as the defensins and cathelicidins. a-defensins secreted by Paneth cells are processed by matrilysin or matrix metalloprotease 7 to form microbicidal peptides. These are regulated through the NOD2 pathway and probiotic bacteria may be important in maintaining Paneth cell secretion of these antimicrobial peptides.[73,74]
Probiotic microorganisms reduce mucosal inflammation in a variety of animal models of inflammatory bowel disease.[71,75,76,77,78,79,80] There are several genetically determined models of colitis, where mice spontaneously or after appropriate stimulation develop colitis, and where probiotics attenuate or prevent colitis. It has been shown that the anti-inflammatory effect of probiotics in experimental colitis may be mediated by stimulation of toll-like receptor 9 by unmethylated CpG DNA of the probiotic bacteria.[81,82] Although this review primarily deals with the intestinal epithelium, it must be recognised that probiotics have effects on sub-epithelial immune cells, including the dendritic cells and T- cells,[83,84] and the effects of probiotics on intestinal immune and other responses must ideally be considered a composite response of the epithelium and sub-epithelial cells. Dendritic cells are antigen-presenting cells found throughout the gut that continually sample enteric antigens and have the ability to discriminate the different microbial strains using the pathogen associated molecular patterns. They then influence naive T cells in the intestine, resulting in T-cell activation and differentiation to Th1 helper, Th2 helper or regulatory Th3 cells.[85] The interaction of probiotic bacteria with dendritic cells results in the upregulation of IL-10 production and decreased production of co-stimulatory molecules and Th1 proinflammatory cytokines. The increase in IL-10 production may have anti-inflammatory effects mediated in part by enhanced numbers of T–regulatory cells.[86] Some studies suggest that probiotic bacteria also upregulate expression of non-epithelial cell molecules involved in host anti-bacterial defense. Probiotic supplementation with strains of Lactobacillus and Bifidobacterium increased the numbers of IgA secreting cells in milk-fed infants.[87,88] Secretory IgA levels have been shown to increase following chronic ingestion of probiotics.[89,90]
Implications of probiotic effects on the epithelium for gastrointestinal disease
Antibiotic-associated diarrhoea and Clostridium difficile diarrhoea:
The normal commensal flora in the lumen of the colon remains in a state of balance, as harmful or pathogenic bacteria are not allowed to flourish in significant numbers in health. The administration of antibiotics (notably ampicillin, amoxicillin, cephalosporins and clindamycin) in the host diminishes or alters the normal colonic flora and leads to antibioticassociated diarrhoea. The pathophysiology of this condition is not fully understood; it has been postulated that the altered fecal flora results in changes in colonic carbohydrate digestion, decreased short-chain fatty acid production and diarrhoea secondary to colonocyte energy depletion.[91] In some patients, there is overgrowth of the pathogen Clostridium difficile, which produces toxins that cause colonic damage. Clostridium difficile is a gram-positive anaerobic bacterium that produces two toxins, an enterotoxin A and a cytotoxin B, which cause colitis.[92,93] Both bacteria and yeast have been studied in the prevention of antibiotic-associated diarrhoea and Clostridium difficile-associated diarrhoea. In a meta-analysis of 10 trials including 1986 children,[94] the per-protocol analysis of 9 showed statistically significant results favoring probiotics (Relative risk 0.49, 95% CI 0.32-0.74) in the prevention of antibiotic-associated diarrhoea, with the number needed to treat to prevent 1 case of diarrhoea being 10. Lactobacillus GG, Bacillus coagulans, and Saccharomyces boulardii were the most effective. On the other hand, probiotics were not so useful in the treatment of C. difficile colitis. Of 4 evaluable studies, only one study showed a statistically significant benefit for probiotics combined with antibiotics while no benefit was seen in the other studies.[95] Although probiotics were not useful in treatment of C. difficile colitis yet their metabolic effects cannot be disregarded. Production of short chain fatty acids may be necessary to regulate colonisation by C. difficile. It may also be important via the effects of SCFA on epithelial cell metabolism, blood flow and differentiation. It is also known that probiotics up-regulate MUC2 and MUC3 gene expression which may be responsible for the reduced colonisation by C. difficile. Acute gastroenteritisProbiotics have been used widely in the prevention and treatment of acute gastroenteritis and acute infectious diarrhoea. A meta-analysis of trials involving prevention of diarrhoea evaluated 34 masked, randomised, controlled trials, and concluded that probiotics reduced the incidence of antibiotic-associated diarrhoea by 52% (95% CI 35-65%), reduced the risk of travellers’ diarrhoea by 8% (-6 to 21%), and that of acute diarrhoea of diverse causes by 34% (8-53%). Probiotics were more effective in preventing acute diarrhoea in children, reducing the risk among children by 57% (35-71%) compared to 26% (7-49%) among adults. The protective effect did not vary significantly among the probiotic strains used.[96] A Cochrane meta-analysis evaluated the role of probiotics in treatment of acute diarrhoea and concluded that mean duration of diarrhoea was shortened by 30.48 hours (18.51-42.46) in patients receiving probiotics.[97] No specific effect of individual probiotic preparations or of diarrhoea 80 Tropical Gastroenterology 2009;30(2):76–85 aetiology was noted. The effects of probiotics on epithelial cell responses to bacterial pathogens have already been described above. The clinical effect of probiotics in iarrhoea may be partly related to the epithelial effects of probiotics and to the effects of probiotics on the intestinal barrier including mucus and antimicrobial peptides, which together contribute towards defense against pathogens.
Inflammatory bowel disease
Animal models of inflammatory bowel disease (IBD) clearly demonstrate a role for endogenous and pathogenic intestinal bacteria in driving the abnormal immune responses. Although mice deficient in the immunoregulatory cytokine interleukin IL-10 develop spontaneous colitis, IL-10 deficient germ-free mice remain disease free.[98] Moreover, feeding specific ‘beneficial’ bacterial strains to these animals reduces inflammatory lesions and modifies cytokine production. The role of endogenous intestinal flora has also been demonstrated in transgenic HLA-B2 rats with colitis,[99] and immune-deficient SCID mice reconstituted with immunocompetent CD45+ T cells.[100] Studies indicate that probiotics can prevent or attenuate experimental colitis in a variety of settings and using a variety of probiotics. Administration of Lactobacillus reuteri significantly reduced inflammation in acetic acid- and methotrexate-induced colitis in rats.[75] Lactobacillus species prevented the development of spontaneous colitis in IL-10 deficient mice101 while continuous feeding with Lactobacillus plantarum was useful in therapy of colitis in the same knockout model.[76] Bifidobacterium infantis and Lactobacillus salivarius were able to attenuate inflammation and reduce the ability to produce Th1-type cytokines in the IL-10 knockout model.[102] Lactobacillus salivarius reduced the rate of progression from inflammation to dysplasia and colonic cancer in IL-10 deficient mice.[103] The probiotic mixture VSL#3 resulted in a significant attenuation of inflammation with a decrease of myeloperoxidase and nitric oxide synthase activity of iodoacetamide-induced colitis.[77]
Although there is evidence of benefit from probiotic use in experimental inflammatory bowel disease, it has been hard to translate this to clinical medicine. Numerous clinical trials have been conducted with probiotics in the management of various situations in inflammatory bowel disease. The trials in inflammatory bowel disease have been conducted in different styles, using different probiotic preparations and very variable end-points that do not allow adequate conclusions regarding the utility of probiotics in IBD. Probiotics have been used to induce remission in ulcerative colitis and have been compared to standard therapy or placebo. Two recent reviews of all available studies found no definite evidence that probiotics were effective in inducing remission in ulcerative colitis.104,105 Formal meta-analysis was not possible due to heterogeneity in the organisms used, the dosages used duration of treatment, and comparators. A number of studies have suggested that administration of a probiotic, nonpathogenic Escherichia coli (Nissle strain 1917), is as effective as standard therapy with aminosalicylates in the maintenance of remission in ulcerative colitis.[106,107,108,109] The data with regard to the use of probiotics in Crohn’s disease is even less complete. A recent Cochrane review of the use of probiotics to induce remission in Crohn’s disease found only one evaluable study with 11 patients.[110] 4 of 5 patients in the probiotic group achieved clinical remission compared to 5 of 6 patients in the placebo group. There was thus insufficient evidence to confirm or exclude a role for probiotics in induction of remission in Crohn’s disease. Three recent reviews, one a Cochrane review, examined the evidence for a role of probiotics in the maintenance of remission in Crohn’s disease.[111,112,113] All three concluded that there was no evidence to support a role for probiotics in the maintenance of remission in patients with Crohn’s disease. The only place in inflammatory bowel disease management where probiotics appear to confer definite benefit is in preventing the onset of acute pouchitis in patients with newly formed ileal pouches, and in maintaining remission following antibacterial treatment of acute pouchitis in patients with a history of refractory or recurrent pouchitis. The benefit is confirmed by a meta-analysis that reviewed 5 trials using the probiotic VSL#3.[114]
Irritable bowel syndrome
The pathogenesis of irritable bowel syndrome remains unknown, and abnormal neural activation, neural innervations, or abnormal innate immune responses have all been implicated. The therapy of irritable bowel syndrome has remained largely unsatisfactory. Against this background, there have been attempts to evaluate a role for probiotics in the therapy of patients with irritable bowel syndrome.
Several recent meta-analyses have examined the evidence for benefit from probiotics in irritable bowel syndrome.[115,116,117,118] In all analyses, it appeared that probiotics were associated with a modest benefit (approximately 20-24%) compared to placebo. These were short term studies, and since irritable bowel syndrome requires long term therapy whether probiotics will provide sustained clinical benefit over longer periods of time, and whether they are useful in specific sub-groups, needs to be evaluated.
Colon cancer
Probiotics and prebiotics alter metabolic processes within the lumen of the gut and within the colonic epithelium, which impact on carcinogenesis.[119,120] Such processes include biotransformation of organic compounds to carcinogens, detoxification of carcinogens, DNA damage and repair in the epithelium, and apoptosis of damaged cells. There is evidence from experimental models showing that aberrant crypt foci are reduced and apoptosis of damaged cells increased after probiotic administration.[121,122,123] Butyrivibrio fibrisolvens, a butyrate-producing bacterium, has been shown to reduce aberrant crypt foci in experimental colon cancer; this organism is found in the feces of apparently healthy rural residents of south India,[124] which may explain a lower incidence of colon cancer in this region. However, clinical trials of probiotic use in the prevention and treatment of colon cancer in humans are scarce or non-existent. This may change in the near future with a resurgence of interest in probiotics.
Probiotics in liver disease
In recent years, it has been recognized that several of the manifestations of chronic liver disease including encephalopathy, endotoxemia and bacterial peritonitis have primary origins in the gut through translocation of molecules or bacteria past the intestinal barrier. Probiotics enhance intestinal barrier function; they also influence innate immune activity and adaptive immunity. Probiotics have therefore been tried in the therapy of chronic liver disease. Small pilot studies or open label studies suggest that probiotics may benefit patients with non-alcoholic steatohepatitis, alcoholic liver disease, and minimal hepatic encephalopathy and lowers endotoxemia in liver cirrhosis.[125,126,127,128,129] These findings need to be confirmed in large randomised trials before a place can be established for the use of probiotics in chronic liver disease.
Conclusion
The interaction of bacteria with the intestinal epithelium has been very well characterised and understood in recent years. The role of probiotic micro-organisms in health and several disease conditions of the gastrointestinal tract in experiments has been detailed in this review. However, their use in clinical medicine in the treatment of gastrointestinal disease is considerably less well established, with clear evidence showing benefit only in antibiotic-associated diarrhoea and acute gastroenteritis. Large studies using standardized protocols along with standardised bacteria types and doses are necessary to conclusively establish a place for probiotics in the management of other gastrointestinal diseases.
References
1. Fuller R. Probiotics in man and animals. J Appl Bacteriol. 1989;66:365–78.
2. Joint FAO/WHO Working Group. Guidelines for the evaluation of probiotics in food. May 2002. http://www.who.int/foodsafety/publications/fs_managementprobiotics2/en
3. Ljungh A, Wadstrom T. Lactic acid bacteria as probiotics. Curr Issues Intest Microbiol. 2006;7:73–89.
4. Balamurugan R, Janardhan HP, George S, Chittaranjan SP, Ramakrishna BS. Bacterial succession in the colon during childhood and adolescence: molecular studies in a southern Indian village. Amer J Clin Nutr. 2008;88:1643–7.
5. Mättö J, Malinen E, Suihko ML, Alander M, Palva A, Saarela M. Genetic heterogeneity and functional properties of intestinal bifidobacteria. J Appl Microbiol. 2004;97:459–70.
6. Bourlioux P, Koletzko B, Guarner F, Braesco V. The intestine and its microflora are partners for the protection of the host: report on the Danone Symposium “The Intelligent Intestine,” held in Paris, June 14, 2002. Am J Clin Nutr. 2003;78:675–83.
7. Balamurugan R, Balaji V, Ramakrishna BS. Faecal carriage of Clostridium difficile in patients with ulcerative colitis using real time polymerase chain reaction. Indian J Med Res. 2008;127:472–7.
8. Balamurugan R, Rajendiran E, George S, Samuel GV, Ramakrishna BS. Real time polymerase chain reaction quantification of specific butyrate-producing bacteria, Desulfovibrios and Enterocococcus faecalis in the faeces of patients with colorectal cancer. J Gastroenterol Hepatol. 2008;23:1298–1303.
9. Zhang M, Liu B, Zhang Y, Wei H, Lei Y, Zhao L. Structural shifts of mucosa-associated lactobacilli and Clostridium leptum subgroup in patients with ulcerative colitis. J Clin Microbiol. 2007;45:496–500.
10. Sokol H, Pigneur B, Watterlot L, Lakhdari O, Bermúdez-Humarán LG, Gratadoux JJ, et al. Faecalibacterium prausnitzii is an antiinflammatory commensal bacterium identified by gut microbiota analysis of Crohn disease patients. Proc Natl Acad Sci U S A. 2008;105:16731–6.
11. Rescigno M. The pathogenic role of intestinal flora in IBD and colon cancer. Curr Drug Targets. 2008;9:395–403.
12. Ramakrishna BS. The normal bacterial flora of the human intestine and its regulation. J Clin Gastroenterol. 2007;41 Suppl 1:S2–6.
13. Goktepe I. Probiotics as biopreservatives for enhancing food safety. IN Probiotics in food safety and human health. Eds. Goktepe I, Juneja VK, Ahmedna M. CRC Press 2006 Boca Raton, FL, USA pp. 285–307.
14. Gibson GR, Wang X. Regulatory effects of bifidobacteria on the growth of other colonic bacteria. J Appl Bacteriol. 1994;77:412–20.
15. Liévin V, Peiffer I, Hudault S, Rochat F, Brassart D, Neeser JR, Servin AL. Bifidobacterium strains from resident infant human gastrointestinal microflora exert antimicrobial activity. Gut 2000;47:646–52.
16. Bernet MF, Brassart D, Neeser JR, Servin AL. Adhesion of human bifidobacterial strains to cultured human intestinal epithelial cells and inhibition of enteropathogen-cell interactions. Appl Environ Microbiol. 1993;59:4121–8.
17. Bernet MF, Brassart D, Neeser JR, Servin AL. Lactobacillus acidophilus LA 1 binds to cultured human intestinal cell lines and inhibits cell attachment and cell invasion by enterovirulent bacteria. Gut. 1994;35:483–9.
18. Muñoz-Provencio D, Llopis M, Antolín M, de Torres I, Guarner F, Pérez-Martínez G, Monedero V. Adhesion properties of Lactobacillus casei strains to resected intestinal fragments and components of the extracellular matrix. Arch Microbiol. 2009;191:153–61.
19. Neeser JR, Granato D, Rouvet M, Servin A, Teneberg S, Karlsson KA. Lactobacillus johnsonii La1 shares carbohydrate-binding specificities with several enteropathogenic bacteria. Glycobiology. 2000;10:1193–9.
20. Boudeau J, Glasser AL, Julien S, Colombel JF, Darfeuille-Michaud A. Inhibitory effect of probiotic Escherichia coli strain Nissle 1917 on adhesion to and invasion of intestinal epithelial cells by adherent-invasive E. coli strains isolated from patients with Crohn’s disease. Aliment Pharmacol Ther. 2000;18:45–56.
21. Fujiwara S, Hashiba H, Hirota T, Forstner JF. Inhibition of the binding of enterotoxigenic Escherichia coli Pb176 to human intestinal epithelial cell line HCT-8 by an extracellular protein fraction containing BIF of Bifidobacterium longum SBT2928: suggestive evidence of blocking of the binding receptor gangliotetraosylceramide on the cell surface. Int J Food Microbiol. 2001;67:97–106.
22. Mattar AF, Teitelbaum DH, Drongowski RA, Yongyi F, Harmon CM, Coran AG. Probiotics up-regulate MUC-2 mucin gene expression in a Caco-2 cell-culture model. Pediatr Surg Int.2002;18:586–90.
23. Kim Y, Kim SH, Whang KY, Kim YJ, Oh S. Inhibition of Escherichia coli O157:H7 attachment by interactions between lactic acid bacteria and intestinal epithelial cells. J Microbiol Biotechnol. 2008;18:1278–85.
24. Caballero-Franco C, Keller K, De Simone C, Chadee K. The VSL#3 probiotic formula induces mucin gene expression and secretion in colonic epithelial cells. Am J Physiol Gastrointest Liver Physiol. 2007;292:G315–22.
25. Mack DR, Ahrne S, Hyde L, Wei S, Hollingsworth MA. Extracellular MUC3 mucin secretion follows adherence of Lactobacillus strains to intestinal epithelial cells in vitro. Gut. 2003;52:827–33
26. Ramakrishna BS, Roediger WE. Bacterial short chain fatty acids: their role in gastrointestinal disease. Dig Dis. 1990;8:337–45.
27. Venkatraman A, Ramakrishna BS, Pulimood A. Butyrate hastens restoration of barrier function after thermal and detergent injury to rat distal colon in vitro. Scand J Gastroenterol. 1999;34:1087–92.
28. Venkatraman A, Ramakrishna BS, Pulimood AB, Patra S, Murthy SN. Increased permeability in dextran sulphate colitis in rats. Time course of development and effect of butyrate. Scand J Gastroenterol. 2000;35:1053–9.
29. Vidyasagar S, Ramakrishna BS. Effects of butyrate on active sodium and chloride transport in rat and rabbit distal colon. J Physiol. 2002;539:163–73.
30. Sakata T, Kojima T, Fujieda M, Takahashi M, Michibata T. Influences of probiotic bacteria on organic acid production by pig caecal bacteria in vitro. Proc Nutr Soc. 2003;62:73–80.
31. Attene-Ramos MS, Wagner ED, Gaskins HR, Plewa MJ. Hydrogen sulphide induces direct radical-associated DNA damage. Mol Cancer Res. 2007;5:455–9.
32. Huycke MM, Abrams V, Moore DR. Enterococcus faecalis produces extracellular superoxide and hydrogen peroxide that damages colonic epithelial cell DNA. Carcinogenesis. 2002;23:529–36.
33. Wang X, Huycke MM. Extracellular superoxide production by Enterococcus faecalis promotes chromosomal instability in mammalian cells. Gastroenterology. 2007;132:551–61.
34. Babidge W, Millard S, Roediger W. Sulfides impair short chain fatty acid beta-oxidation at acyl-CoA dehydrogenase level in colonocytes: implications for ulcerative colitis. Mol Cell Biochem. 1998;181:117–24.
35. Ramakrishna BS, Gee D, Weiss A, Pannall P, Roberts-Thomson IC, Roediger WE. Estimation of phenolic conjugation by colonic mucosa. J Clin Pathol. 1989;42:620–3.
36. Ramakrishna BS, Roberts-Thomson IC, Pannall PR, Roediger WE. Impaired conjugation of phenols by the colonic mucosa in active and quiescent ulcerative colitis. Gut. 1991;32:46–9.
37. De Preter V, Vanhoutte T, Huys G, Swings J, Rutgeerts P, Verbeke K. Effect of lactulose and Saccharomyces boulardii administration on the colonic urea-nitrogen metabolism and the bifidobacteria concentration in healthy human subjects. Aliment Pharmacol Ther. 2006;23:963–74.
38. Nicaise C, Prozzi D, Viaene E, Moreno C, Gustot T, Quertinmont E, et al. Control of acute, chronic, and constitutive hyperammonemia by wild-type and genetically engineered Lactobacillus plantarum in rodents. Hepatology. 2008;48:1184–92.
39. Seth A, Yan F, Polk DB, Rao RK. Probiotics ameliorate the hydrogen peroxide-induced epithelial barrier disruption by a PKC- and MAP kinase-dependent mechanism. Am J Physiol Gastrointest Liver Physiol. 2008;294:G1060–9.
40. Johnson-Henry KC, Donato KA, Shen-Tu G, Gordanpour M, Sherman PM. Lactobacillus rhamnosus strain GG prevents enterohemorrhagic Escherichia coli O157:H7-induced changes in epithelial barrier function. Infect Immun. 2008;76:1340–8.
41. Putaala H, Salusjärvi T, Nordström M, Saarinen M, Ouwehand AC, Bech Hansen E, et al. Effect of four probiotic strains and Escherichia coli O157:H7 on tight junction integrity and cyclooxygenase expression. Res Microbiol. 2008;159:692–8.
42. Ewaschuk JB, Diaz H, Meddings L, Diederichs B, Dmytrash A, Backer J, et al. Secreted bioactive factors from Bifidobacterium infantis enhance epithelial cell barrier function. Am J Physiol Gastrointest Liver Physiol. 2008;295:G1025–34.
43. Zyrek AA, Cichon C, Helms S, Enders C, Sonnenborn U, Schmidt MA. Molecular mechanisms underlying the probiotic effects of Escherichia coli Nissle 1917 involve ZO-2 and PKC zeta redistribution resulting in tight junction and epithelial barrier repair. Cell Microbiol. 2007;9:804–16.
44. Madsen K, Cornish A, Soper P, McKaigney C, Jijon H, Yachimec C, et al. Probiotic bacteria enhance murine and human intestinal epithelial barrier function. Gastroenterology. 2001;121:580–91.
45. Mazmanian SK, Liu CH, Tzianabos AO, Kasper DL. An immunomodulatory molecule of symbiotic bacteria directs maturation of the host immune system. Cell. 2005;122:107–18.
46. Tubelius P, Stan V, Zachrisson A. Increasing work-place healthiness with the probiotic Lactobacillus reuteri: a randomised, double-blind placebo-controlled study. Environ Health. 2005;4:25.
47. Weizman Z, Asli G, Alsheikh A. Effect of a probiotic infant formula on infections in child care centers: comparison of two probiotic agents. Pediatrics. 2005;115:5–9.
48. de Vrese M, Winkler P, Rautenberg P, Harder T, Noah C, Laue C, et al. Probiotic bacteria reduced duration and severity but not the incidence of common cold episodes in a double blind, randomized, controlled trial. Vaccine. 2006;24:6670–4.
49. Mason KL, Huffnagle GB, Noverr MC, Kao JY. Overview of gut immunology. Adv Exp Med Biol. 2008;635:1–14.
50. Michelsen KS, Arditi M. Toll-like receptors and innate immunity in gut homeostasis and pathology. Curr Opin Hematol. 2007;14:48–54.
51. Fagarasan S, Honjo T. Intestinal IgA synthesis: regulation of frontline body defences. Nat Rev Immunol. 2003;3:63–72.
52. Sudo N, Sawamura S, Tanaka K, Aiba Y, Kubo C, Koga Y. The requirement of intestinal bacterial flora for the development of an IgE production system fully susceptible to oral tolerance induction. J Immunol. 1997;159:1739–45.
53. Hoarau C, Lagaraine C, Martin L, Velge-Roussel F, Lebranchu Y. Supernatant of Bifidobacterium breve induces dendritic cell maturation, activation, and survival through a Toll-like receptor 2 pathway. J Allergy Clin Immunol. 2006;117:696–702.
54. Müller U, Vogel P, Alber G, Schaub GA. The innate immune system of mammals and insects. Contrib Microbiol. 2008;15:21–44.
55. Akira S. Toll-like receptor signaling. J Biol Chem. 2003;278:38105–8.
56. Jung HC, Eckmann L, Yang SK, Panja A, Fierer J, Morzycka-Wroblewska E, Kagnoff MF. A distinct array of proinflammatory cytokines is expressed in human colon epithelial cells in response to bacterial invasion. J Clin Invest. 1995;95:55–65.
57. Rodríguez BL, Rojas A, Campos J, Ledon T, Valle E, Toledo W, Fando R. Differential interleukin-8 response of intestinal epithelial cell line to reactogenic and nonreactogenic candidate vaccine strains of Vibrio cholerae. Infect Immun. 2001;69:613–6.
58. Sansonetti PJ. Rupture, invasion and inflammatory destruction of the intestinal barrier by Shigella, making sense of prokaryoteeukaryote cross-talks. FEMS Microbiol Rev. 2001;25:3–14.
59. Nandakumar NS, Srinivasan P, Mohan KM, Jayakanthan K, Ramakrishna BS. Effect of Vibrio cholerae on chemokine gene expression in HT29 cells and its modulation by Lactobacillus GG. Scand J Immunol. 2009 (In press).
60. Casola A, Garofalo RP, Crawford SE, Estes MK, Mercurio F, Crowe SE, et al. Interleukin-8 gene regulation in intestinal epithelial cells infected with rotavirus: role of viral-induced IkappaB kinase activation. Virology. 2002;298:8–19.
61. O’Hara AM, O’Regan P, Fanning A, O’Mahony C, Macsharry J, Lyons A, et al. Functional modulation of human intestinal epithelial cell responses by Bifidobacterium infantis and Lactobacillus salivarius. Immunology. 2006;118:202–15.
62. Otte JM, Podolsky DK. Functional modulation of enterocytes by gram-positive and gram-negative microorganisms. Am J Physiol Gastrointest Liver Physiol. 2004;286:G613–26.
63. Tien MT, Girardin SE, Regnault B, Le Bourhis L, Dillies MA, Coppée JY, et al. Anti-inflammatory effect of Lactobacillus casei on Shigella-infected human intestinal epithelial cells. J Immunol. 2006;176:1228–37.
64. Broekaert IJ, Nanthakumar NN, Walker WA. Secreted probiotic factors ameliorate enteropathogenic infection in zinc-deficient human Caco-2 and T84 cell lines. Pediatr Res. 2007;62:139–44.
65. Corr SC, Gahan CG, Hill C. Impact of selected Lactobacillus and Bifidobacterium species on Listeria monocytogenes infection and the mucosal immune response. FEMS Immunol Med Microbiol. 2007;50:380–8.
66. Riedel CU, Foata F, Philippe D, Adolfsson O, Eikmanns BJ, Blum S. Anti-inflammatory effects of bifidobacteria by inhibition of LPSinduced NF-kappa B activation. World J Gastroenterol. 2006;12:3729–35.
67. AM, O’Regan P, Fanning A, O’Mahony C, Macsharry J, Lyons A, et al. Functional modulation of human intestinal epithelial cell responses by Bifidobacterium infantis and Lactobacillus salivarius. Immunology. 2006;118:202–15.
68. Mattar AF, Teitelbaum DH, Drongowski RA, Yongyi F, Harmon CM, Coran AG. Probiotics up-regulate MUC-2 mucin gene expression in a Caco-2 cell-culture model. Pediatr Surg Int. 2002;18:586–90.
69. Bai AP, Ouyang Q, Xiao XR, Li SF. Probiotics modulate inflammatory cytokine secretion from inflamed mucosa in active ulcerative colitis. Int J Clin Pract. 2006;60:284–8.
70. Borruel N, Carol M, Casellas F, Antolín M, de Lara F, Espín E, et al. Increased mucosal tumour necrosis factor alpha production in Crohn’s disease can be downregulated ex vivo by probiotic bacteria. Gut. 2002;51:659–64.
71. Nandakumar NS, Balamurugan R, Jayakanthan K, Pulimood A, Pugazhendhi S, Ramakrishna BS. Probiotic administration alters the gut flora and attenuates colitis in mice administered dextran sodium sulfate. J Gastroenterol Hepatol. 2008;23:1834–9.
72. Hoffmann M, Rath E, Hölzlwimmer G, Quintanilla-Martinez L, Loach D, Tannock G, et al. Lactobacillus reuteri 100-23 transiently activates intestinal epithelial cells of mice that have a complex microbiota during early stages of colonization. J Nutr. 2008;138:1684–91.
73. Wehkamp J, Fellermann K, Stange EF. Human defensins in Crohn’s disease. Chem Immunol Allergy. 2005;86:42–54.
74. Newburg DS, Walker WA. Protection of the neonate by the innate immune system of developing gut and of human milk. Pediatr Res. 2007;61:2–8.
75. Fabia R, Ar’Rajab A, Johansson ML, Willén R, Andersson R, Molin G, et al. The effect of exogenous administration of Lactobacillus reuteri R2LC and oat fiber on acetic acid-induced colitis in the rat. Scand J Gastroenterol. 1993;28:155–62.
76. Schultz M, Veltkamp C, Dieleman LA, Grenther WB, Wyrick PB, Tonkonogy SL, et al. Lactobacillus plantarum 299V in the treatment and prevention of spontaneous colitis in interleukin-10-deficient mice. Inflamm Bowel Dis. 2002;8:71–80.
77. Shibolet O, Karmeli F, Eliakim R, Swennen E, Brigidi P, Gionchetti P, et al. Variable response to probiotics in two models of experimental colitis in rats. Inflamm Bowel Dis. 2002;8:399–406.
78. Mennigen R, Nolte K, Rijcken EM, Utech M, Loeffler B, Senninger N, Bruewer M. Probiotic mixture VSL#3 protects the epithelial barrier by maintaining tight junction protein expression and preventing apoptosis in a murine model of colitis. Am J Physiol Gastrointest Liver Physiol. 2009 Feb 12. [Epub ahead of print]
79. van der Kleij H, O’Mahony C, Shanahan F, O’Mahony L, Bienenstock J. Protective effects of Lactobacillus reuteri and Bifidobacterium infantis in murine models for colitis do not involve the vagus nerve. Am J Physiol Regul Integr Comp Physiol. 2008;295:R1131–7.
80. Amit-Romach E, Uni Z, Reifen R. Therapeutic potential of two probiotics in inflammatory bowel disease as observed in the trinitrobenzene sulfonic acid model of colitis. Dis Colon Rectum. 2008;51:1828–36.
81. Lee J, Rachmilewitz D, Raz E. Homeostatic effects of TLR9 signaling in experimental colitis. Ann N Y Acad Sci. 2006;1072:351–5.
82. Rachmilewitz D, Katakura K, Karmeli F, Hayashi T, Reinus C, Rudensky B, et al. Toll-like receptor 9 signaling mediates the antiinflammatory effects of probiotics in murine experimental colitis. Gastroenterology. 2004;126:520–8.
83. Drakes M, Blanchard T, Czinn S. Bacterial probiotic modulation of dendritic cells. Infect Immun. 2004;72:3299–309.
84. Hart AL, Lammers K, Brigidi P, Vitali B, Rizzello F, Gionchetti P, et al. Modulation of human dendritic cell phenotype and function by probiotic bacteria. Gut. 2004;53:1602–9.
85. Kaliñski P, Hilkens CM, Wierenga EA, Kapsenberg ML. T-cell priming by type-1 and type-2 polarized dendritic cells: the concept of a third signal. Immunol Today. 1999;20:561–7.
86. Christensen HR, Frøkiaer H, Pestka JJ. Lactobacilli differentially modulate expression of cytokines and maturation surface markers in murine dendritic cells. J Immunol. 2002;168:171–8.
87. Rinne M, Kalliomaki M, Arvilommi H, Salminen S, Isolauri E. Effectof robiotics and breastfeeding on the bifidobacterium ndlactobacillus/enterococcus microbiota and humoral immuneresponses. J ediatr. 2005;147:186–91.
88. Rautava S, Arvilommi H, Isolauri E. Specific probiotics in enhancingmaturation of IgA responses in formula-fed infants. Pediatr Res.2006;60:221–4.
89. Perdigón G, Alvarez S, Pesce de Ruiz Holgado A. Immunoadjuvantactivity of oral Lactobacillus casei: influence of dose on thesecretory immune response and protective capacity in intestinalinfections. J Dairy Res. 1991;58:485–96.
90. Marteau P, Vaerman JP, Dehennin JP, Bord S, Brassart D, PochartP, et al. Effects of intrajejunal perfusion and chronic ingestion ofLactobacillus johnsonii strain La1 on serum concentrations and jejunal secretions of immunoglobulins and serum proteins inhealthy humans. Gastroenterol Clin Biol. 1997;21:293–8.
91. Clausen MR. Production and oxidation of short-chain fatty acidsin the human colon: implications for antibiotic-associated diarrhea,ulcerative colitis, colonic cancer, and hepatic encephalopathy.Dan Med Bull. 1998;45:51–75.
92. McFarland LV. Antibiotic-associated diarrhea: epidemiology,trends and treatment. Future Microbiol. 2008;3:563–78.
93. Navaneethan U, Giannella RA. Mechanisms of infectiousdiarrhea. Nat Clin Pract Gastroenterol Hepatol.2008;5:637–47.
94. Johnston BC, Supina AL, Ospina M, Vohra S. Probiotics for theprevention of pediatric antibiotic-associated diarrhea. CochraneDatabase Syst Rev. 2007;(2):CD004827.
95. Pillai A, Nelson R. Probiotics for treatment of Clostridium difficile-associated colitis in adults. Cochrane Database Syst Rev. 2008;(1): CD004611.
96. Sazawal S, Hiremath G, Dhingra U, Malik P, Deb S, Black RE.Efficacy of probiotics in prevention of acute diarrhoea: a meta-analysis of masked, randomised, placebo-controlled trials. LancetInfect Dis. 2006;6:374–82.
97. Allen SJ, Okoko B, Martinez E, Gregorio G, Dans LF. Probioticsfor treating infectious diarrhoea. Cochrane Database Syst Rev.2004; (2): CD003048.
98. Sellon RK, Tonkonogy S, Schultz M, Dieleman LA, Grenther W,Balish E, et al. Resident enteric bacteria are necessary fordevelopment of spontaneous colitis and immune system activationin interleukin-10-deficient mice. Infect Immun.1998;66:5224–31.
99. Taurog JD, Richardson JA, Croft JT, Simmons WA, Zhou M,Fernández-Sueiro JL, et al. The germfree state preventsdevelopment of gut and joint inflammatory disease in HLA-B27transgenic rats. J Exp Med. 1994;180:2359–64.
100.Morrissey PJ, Charrier K, Horovitz DA, Fletcher FA, Watson JD.Analysis of the intra-epithelial lymphocyte compartment in SCIDmice that received co-isogenic CD4+ T cells. Evidence that maturepost-thymic CD4+ T cells can be induced to express CD8 alphain vivo. J Immunol. 1995;154:2678–86.
101.Madsen KL, Doyle JS, Jewell LD, Tavernini MM, Fedorak RN.Lactobacillus species prevents colitis in interleukin 10 gene-deficient mice. Gastroenterology. 1999;116:1107–14.
102.McCarthy J, O’Mahony L, O’Callaghan L, Sheil B, Vaughan EE,Fitzsimons N, et al. Double blind, placebo controlled trial of twoprobiotic strains in interleukin 10 knockout mice and mechanisticlink with cytokine balance. Gut 2003;52:975–80.
103.O’Hara AM, O’Regan P, Fanning A, O’Mahony C, Macsharry J,Lyons A, et al. Functional modulation of human intestinal epithelialcell responses by Bifidobacterium infantis and Lactobacillussalivarius. Immunology. 2006;118:202–15.
104.Mallon P, McKay D, Kirk S, Gardiner K. Probiotics for induction ofremission in ulcerative colitis. Cochrane Database Syst Rev.2007; (4): CD005573.
105.Zigra PI, Maipa VE, Alamanos YP. Probiotics and remission ofulcerative colitis: a systematic review. Neth J Med.2007;65:411–8.
106.Kruis W, Schütz E, Fric P, Fixa B, Judmaier G, Stolte M. Double-blind comparison of an oral Escherichia coli preparation andmesalazine in maintaining remission of ulcerative colitis. AlimentPharmacol Ther. 1997;11:853–8.
107.Rembacken BJ, Snelling AM, Hawkey PM, Chalmers DM, AxonAT. Non-pathogenic Escherichia coli versus mesalazine for thetreatment of ulcerative colitis: a randomised trial. Lancet.1999;354:635–9.
108.Kruis W, Fric P, Pokrotnieks J, Lukás M, Fixa B, Kascák M, et al.Maintaining remission of ulcerative colitis with the probioticEscherichia coli Nissle 1917 is as effective as with standardmesalazine. Gut. 2004;53:1617–23.
109.Henker J, Müller S, Laass MW, Schreiner A, Schulze J. ProbioticEscherichia coli Nissle 1917 (EcN) for successful remissionmaintenance of ulcerative colitis in children and adolescents: anopen-label pilot study. Z Gastroenterol. 2008;46:874–5.
110.Butterworth AD, Thomas AG, Akobeng AK. Probiotics for inductionof remission in Crohn’s disease. Cochrane Database Syst Rev.2008; (3): CD006634.
111.Rolfe VE, Fortun PJ, Hawkey CJ, Bath-Hextall F. Probiotics formaintenance of remission in Crohn’s disease. Cochrane DatabaseSyst Rev. 2006; (4): CD004826.
112.Rahimi R, Nikfar S, Rahimi F, Elahi B, Derakhshani S, Vafaie M, etal. A meta-analysis on the efficacy of probiotics for maintenanceof remission and prevention of clinical and endoscopic relapsein Crohn’s disease. Dig Dis Sci. 2008;53:2524–31.
113.Akobeng AK. Review article: the evidence base for interventionsused to maintain remission in Crohn’s disease. Aliment PharmacolTher. 2008;27:11–8.
114.Elahi B, Nikfar S, Derakhshani S, Vafaie M, Abdollahi M. On thebenefit of probiotics in the management of pouchitis in patientsunderwent ileal pouch anal anastomosis: a meta-analysis ofcontrolled clinical trials. Dig Dis Sci. 2008;53:1278–84.
115.Moayyedi P, Ford AC, Talley NJ, Cremonini F, Foxx-Orenstein A,Brandt L, et al. The efficacy of probiotics in the therapy of irritablebowel syndrome: a systematic review. Gut. 2008 Dec 17. [Epubahead of print]
116.Nikfar S, Rahimi R, Rahimi F, Derakhshani S, Abdollahi M. Efficacyof probiotics in irritable bowel syndrome: a meta-analysis ofrandomized, controlled trials. Dis Colon Rectum.2008;51:1775–80.
117.McFarland LV, Dublin S. Meta-analysis of probiotics for thetreatment of irritable bowel syndrome. World J Gastroenterol.2008;14:2650–61.
118.Hoveyda N, Heneghan C, Mahtani KR, Perera R, Roberts N,Glasziou P. A systematic review and meta-analysis: Probioticsin the treatment of irritable bowel syndrome. BMC Gastroenterol.2009;9:15.
119.Wollowski I, Rechkemmer G, Pool-Zobel BL. Protective role ofprobiotics and prebiotics in colon cancer. Am J Clin Nutr.2001;73:451S–455S.
120.de Moreno de LeBlanc A, Matar C, Perdigón G. The application of probiotics in cancer. Br J Nutr. 2007;98 Suppl 1:S105–10.
121.Geier MS, Butler RN, Howarth GS. Probiotics, prebiotics andsynbiotics: a role in chemoprevention for colorectal cancer?Cancer Biol Ther. 2006;5:1265–9.
122.Ohkawara S, Furuya H, Nagashima K, Asanuma N, Hino T. Oraladministration of Butyrivibrio fibrisolvens, a butyrate-producingbacterium, decreases the formation of aberrant crypt foci in thecolon and rectum of mice. J Nutr. 2005;135:2878–83.
123.Le Leu RK, Brown IL, Hu Y, Bird AR, Jackson M, Esterman A, etal. A synbiotic combination of resistant starch and Bifidobacteriumlactis facilitates apoptotic deletion of carcinogen-damaged cellsin rat colon. J Nutr. 2005;135:996–1001.
124.Balamurugan R, Aarthi CM, Chittaranjan SP, Ramakrishna BS.Molecular detection of the ruminal bacterium, Butyrivibriofibrisolvens, in feces from rural residents of southern India.Microbial Ecol Health Dis. 2009 (In press).
125.Lirussi F, Mastropasqua E, Orando S, Orlando R. Probiotics fornon-alcoholic fatty liver disease and/or steatohepatitis. CochraneDatabase Syst Rev. 2007; (1): CD005165.
126.Lata J, Novotný I, Príbramská V, Juránková J, Fric P, Kroupa R, etal. The effect of probiotics on gut flora, level of endotoxin andChild-Pugh score in cirrhotic patients: results of a double-blindrandomized study. Eur J Gastroenterol Hepatol.2007;19:1111–3.
127.Sharma P, Sharma BC, Puri V, Sarin SK. An open-label randomizedcontrolled trial of lactulose and probiotics in the treatment ofminimal hepatic encephalopathy. Eur J Gastroenterol. Hepatol.2008;20:506–11.
128.Bajaj JS, Saeian K, Christensen KM, Hafeezullah M, Varma RR,Franco J, et al. Probiotic yogurt for the treatment of minimalhepatic encephalopathy. Am J Gastroenterol.2008;103:1707–15.
129.Kirpich IA, Solovieva NV, Leikhter SN, Shidakova NA, LebedevaOV, Sidorov PI, et al. Probiotics restore bowel flora and improveliver enzymes in human alcohol-induced liver injury: a pilot study.Alcohol. 2008;
|